SkyDude
Astronomy
TECHNIQUES
Image-train
for TS-Optics 130 with ATREDT30
TS-Optics 130
ATREDT30
8 mm spacer
0.4 mm spacer
Camera Assembly (see below)
Image-train
for TS-Optics 130 with AT2FF
TS-Optics 130
AT2FF
5 mm spacer
Camera Assembly (see below)
Image-train for SV70T with
SFFR-70APO
SV70T
SFFR-70APO
8 mm spacer
0.4 mm spacer
Camera Assembly (see below)
Image-train
for Camera Assembly
Orion Thin OAG
8 mm spacer, filed down to
about 7.8 mm *
ZWO Filter Wheel
0.1 mm spacer **
ZWO ASI1600 Pro Camera
* The 8 mm spacer was filed down to get the correct OAG position,
rotation-wise to the camera (OAG screws in a bit more so the pick-off
prism
lines up with the top of the camera sensor).
** The 0.1 mm spacer is to get the
correct camera position
rotation-wise on the filter wheel (camera is screwed in a bit
less so the top of the camera lines up with
the top of the filter wheel).
Fine-tuning
the Spacing
Most telescope designs result in "off-axis
aberrations", which is a fancy way of saying that the stars at the edge
of the field-of-view are not round. For reflectors, this is
called
"coma", where the stars look like comets. For refractors, this
is
called "field curvature", and the stars look "pointy", which means
elongated toward and away from the center of the image. Coma can be
corrected by using a coma-corrector, while field curvature can
be
corrected by using a Field-Flattener or a combination
Field-Flattener/Focal-Reducer. All of these require proper
spacing
between the corrector and the camera sensor. A very common spacing
requirement is 55 mm.
For best results the spacing should be
fine-tuned. This can be a long and tedious process: take an image, look
at the corner stars, adjust the spacing, repeat. Here are some
things that may be helpful:
- Scopes with short
focal-lengths have more field curvature.
- Faster scopes (lower F-ratio)
make field
curvature more visible, because more light rays are
out of focus.
- Spacing is much more critical
with Focal-Reducer/Field-Flatteners than with straight Field-Flatteners.
- Start at 55 mm or recommended
spacing.
- Increase
spacing if the corner stars look "pointy", decrease if they look "flat"
or have a funny "inside out" appearance from too much
correction.
The
worst-case scenario would be a short fast scope using a
Focal-Reducer/Field-Flattener, as in the case of the SV70T with the
SFFR-70APO, where a spacing change of 0.1 mm makes a small but
noticeable difference. Best case scenario would be a long slow
refractor (say 1200 mm and F10) where a corrector may not even be
needed. More forgiving as well is a straight Field-Flattener. With the
TS-Optics 130 and AT2FF, spacing can be off by +/- 2 mm and still have
good results.
An unexpected characteristic I've
seen is that the rotational position of a
corrector seems
to have an impact as well. I first saw this while
fine tuning
the SV70T with the SFFR-70APO. When I got close to perfect spacing
with corners stars almost perfectly round, I noticed
that the
stars were "out of balance". That is, the stars in one
corner looked slightly different than the opposite corner. I
continued to adjust the spacing in tiny amounts, +/- 0.1 mm, until the
stars were as balanced as possible. But note that adjusting in those
small increments doesn't change the spacing much, but it does change
the rotational position of the corrector. My theory is that both the
corrector and scope
have a tiny bit of optical tilt., so depending on
the rotational
position of the two, the tilts tend to exaggerate or cancel
each other.
AT8RC
Collimation
It's easy to tell when a Ritchy-Chretien
scope is out of collimation. The tell-tale sign is oblong
stars at
one side of the image while the other side looks better. You may also
notice a slight coma-flare on stars, most noticeable at the center of
the image.
But collimating an Astro-Tech
Ritchy-Chretien scope can be a daunting experience for the
following reasons:
- The collimation instructions
in
the manual are not good for imaging and can actually make things worse.
- The same goes when attempting
to use the standard way of collimating a reflector or SCT.
- There is a lot of misleading
info on the internet (nawh!)
- The adjustments are not
intuitive.
After much frustration, I
finally embarked on a step-by-step experiment and discovered
the following:
Secondary Mirror:
- Adjusting the secondary mirror
mainly affects the oblong stars at the edge of the image.
- Tightening a secondary
mirror screw (and loosening the
other 2
screws) reduces star elongation of the corresponding edge.
- This is best seen by viewing
an in-focus image of at least 1 minute exposure time.
Primary Mirror:
- Adjusting the primary mirror
mainly affects the coma of stars in the image.
- When de-focused OUTWARD,
loosening
a primary mirror black-screw (and tightening its
mating
silver-screw) reduces the coma flare or "fat" of the
corresponding
side of the star-donut. This also reduces the
brightness of the other side of the donut.
- This is best seen by viewing a
slightly out of focus star-donut at the center of the image.
Armed with this info, I
arrived at the following procedure:
Preparation:
- First
try using a proven field-flattener such as the Astro-Tech ATFF2 or the
Astro-Physics CCDT67. The results may be so good you'll forget all
about collimating!
- Aim the scope
toward a suitable field of stars such as a large bright open
cluster.
- Attach the camera and guiding
setup.
- If
you use a field-flattener or focal reducer, it's important to attach it
as well because they seem to shift the field
curvature as
well as flatten it, hence requiring different adjustments.
- Rotate
the camera so that the top of the image corresponds with the top of the
scope. For DSLRs, the top of the camera should be aligned with the
bottom
of the scope. For most CCDs, the top should be aligned 90 deg
to the right. (CCD
cameras are designed this way so that Dec movements correspond to
up/down on the image.)
- Confirm
the rotation by de-focusing OUTWARD, taking an image
with your hand in front of
the top part of the scope, and looking for a gap on the top
part of
the star-donuts.
Warning1: If you de-focus
inward, the donut gap will be on the bottom (opposite of where you
placed your hand).
Warning2: Failure to confirm the rotation may result
in adjusting the wrong screw!
Primary Mirror Collimation:
- Using the
focus mode of your software, de-focus OUTWARD slightly so that the
stars are very tiny donuts.
- Select
a star near the center of the image, and determine which side has flare
or is fatter. The other side will be brighter, which is sometimes
easier
to see.
- Loosen
the primary black-screw that corresponds to the flare by no more than
1/16 of a turn and tighten its mating silver-screw.
- Repeat
steps 1-3 until the star-donut is balanced.
Secondary Mirror Collimation:
- Focus the
scope, switch on guiding, and take a 1-inute image.
- Examine
the corner stars and choose the corner with the most oblong stars
(stars stretched toward and away from the center of the image). If you
are using a field-flattener, this may require some careful
examination to determine the worst corner.
- Tighten
the corresponding secondary screw by no more than 1/16 of a turn and
loosen the other screws by the same amount.
- Repeat
steps 1-3 until each corner is balanced with its opposite
corner. Don't be surprised if
one set of opposite corners has more elongation than the other set.
This is normal for a Ritchy-Chretien.
Note1: If at any time the stars
look like triangles, the secondary mirror is pinched. Loosening all 3
screws will fix it.
Note2:
If you are not using a field-flattener, you may notice the secondary
adjustment
causes the center stars to become slightly oblong. Back off the
adjustment a bit to reach a happy compromise.
After collimation
is complete, and assuming you have one of the above mentioned
field-flatteners, you should have well shaped stars across the
entire image.
Example Images:
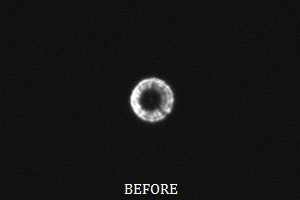
Primary Collimation: The
right side of the donut is flared and the left is brighter. Loosen
the right-hand black-screw and tighten its mating silver-screw.
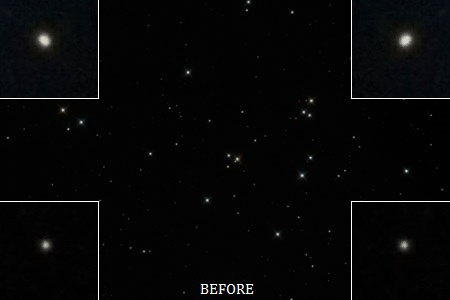
Secondary
Collimation: The stars at the top are the most elongated.
Tighten
the top screw and loosen the other 2 screws.
The good folks at Deep Sky
Instruments offer a similar collimation procedure and some great info
on Ritchy-Chretiens.
The main difference between our methods is that I found it is easier to
see oblong edge-stars on an image that is
in-focus,
rather than out-of-focus:
Another Astro-Tech RC user with a similar method, and great images:
http://astroimages.weebly.com/collimating-an-at8rc.html
Off-axis-guider
(OAG) vs. Guide Scope
Back in the days of film imaging and manual guiding this was a great
controversy. A guide-scope system was much easier to use but would
typically have
flexure problems that were difficult or even impossible to solve. OAGs
have no flexure, but finding a guide-star was sometimes impossible, and
guiding was
sometimes too difficult to yield good results. I used an OAG system
when I used to image with film.
But now we have digital cameras, computers, and specialized software.
The result is that guide-stars are selected by point-and-click, mounts
are guided automatically, and sub-frame exposure times are typically 5
minutes, which in most setups would not be long enough to present
flexure problems. Modern guide cameras such as the
Starlight Xpress LodeStar or the QHYCCD QHY5L-IIM are so sensitive they
can be used on an OAG with little
difficulty in finding guide-stars. The decision between OAG or
guide-scope is less of a
controversy and more a matter of choice.
But oblong stars are still reported by guide-scope users, even
when they image with a refractor (fixed optics) and have a very solid
setup. I suspect that often overlooked is
the fact
that the guide-scope
focal length is too short. There is a tendency these days to use short
focal length guide-scopes, even finder-scopes, which degrades the
ability of the software to calculate star position.
Through testing I have demonstrated the following equation in regard
to the guide-scope and guide-camera:
Guiding Accuracy =
Guide-scope-focal-length * Guide-star-image-quality
Yes, guiding software is capable of sub-pixel guiding down to 1/10
pixel, but that is
only true if the star-image quality is large enough and good enough for
the software to
calculate the guide-star position accurately. Poor image = poor
guiding, and you may not get a star image that is good enough when
using a very short focal-length guide-scope.
If using a guide-scope:
Avoid guide-scope
focal-lengths that are too short - no less than 1/4th of the imaging
focal-length.
The longer the guide-scope
focal length - the better.
The more sensitive the guide
camera - the better.
Try to keep sub-frame exposure
times to 5 minutes or less.
Understand that guiding accuracy may suffer when a dim guide-star is
selected.
If using an OAG, or the new On-Axis-Guider from Innovations Foresight
(see Links):
Use a very sensitive guide
camera, such as the LodeStar or the QHY5L-IIM (see Links).
Sub-frame exposure times may
be as long as you want.
Polar Alignment
I am about to enter a perilous area of controversy by stating the
following:
I think in many cases the importance of precise polar-alignment is over
emphasized. (gasp!)
Back in the days of imaging with film, polar alignment was very
important. Exposure times were an hour or longer, and guide-scopes
might have to be pointed a few degrees away from the center of the
image in order to point to a bright enough guide-star. The combination
of the two could result in stars that were shaped like an arc, unless
the mount was perfectly polar-aligned.
But with digital imaging and guide cameras, typical exposure times are
5 minutes, and guide-scopes can remain at the same aim as the imaging
camera. The importance of polar-alignment has been greatly
reduced. I've even heard arguments that a small amount of
polar-misalignment is better since it causes the mount to always
perform DEC corrections in one direction, and avoid backlash problems.
There is also the idea that a slight amount of
polar-misalignment
causes a little bit of dithering between images, and so reduces
fixed-pattern-noise. For me, I just use the mount's polar-alignment
scope. The typical accuracy of less than 0.25 degree
it
provides is good enough for most
imaging.
But there are 2 important exceptions to this rule:
1. When imaging without any
guiding at all, fairly precise polar-alignment is needed to keep the
frames reasonably similar.
2. When imaging an object very
close to the poles (10 deg or so), the mount may not be able to keep up
with the needed R/A corrections. This occurs because DEC corrections
cause increasingly larger R/A movements as the you get closer to the
poles. Precise polar alignment keeps this problem to a minimum.
Comments
welcome: mark.eby@twc.com